|
2.8 The fungal phylogeny
A global phylogeny of fungi first emerged using data from
several gene regions: 18S rRNA, 28S rRNA, 5.8S rRNA, elongation factor-1 (EF1),
and RNA polymerase II subunits (RPB1 and RPB2). Data for all these gene regions
were combined (a total number of 6,436 aligned nucleotides) for 199 fungi (James
et al., 2006), and there have been several more genome level studies
since this work was published, many of which were combined by McCarthy &
Fitzpatrick (2017). The outcome is an enormous cladogram which we can’t
reproduce here, but a highly simplified evolutionary tree is shown in Fig. 10
below.
Significantly, this extensive analysis generally supports
the more traditional arrangement into: Ascomycota,
Basidiomycota, various zygomycetes and Chytridiomycota,
but it obviously adds new detail to that traditional structure. Ascomycota
and Basidiomycota are united as the Dikarya, fungi in which
at least part of the life cycle is characterised by cells with paired
nuclei. The closest relatives of these two sister groups are the
Glomeromycotina (which was for a long time included as the Glomales
within the ‘Zygomycota’). Neither the ‘Zygomycota’ nor the
Chytridiomycota are monophyletic groups; they have representatives
in different clades or branches of the tree that are grouped into those
phyla by their shared primitive morphologies (such groups are called
paraphyletic). This is why, in the latest classification (see Chapter 3) the
Chytridiomycota is redefined and, as mentioned above, the ‘Zygomycota’ is
demoted from rank as a formal taxon and becomes an informal name, at least
for the time being.
|
Fig. 10. The main branches of the
tree of life for Kingdom Fungi, as established by the AFTOL project and
subsequent publications (referenced in the text). All the traditional phyla
are represented: Ascomycota, Basidiomycota, zygomycetes (as Mucoromycota,
which includes Glomeromycotina, and Zoopagomycota), and Chytridiomycota (see
discussion in the text of this chapter and Chapter 3 for Blastocladiomycota
and Neocallimastigomycota). Ascomycota and Basidiomycota are united as the
Dikarya, fungi in which at least part of the life cycle is characterised by
cells with paired nuclei (‘dikaryons’). The closest relatives of these two
sister groups are the Glomeromycotina. The multigene phylogeny of fungi
established by the AFTOL project placed the Glomeromycotina (using the name
Glomeromycota) in a basal position as a sister group of Asco- and
Basidiomycota (together called subkingdom Dikarya); this relationship to
Dikarya is only slightly changed by the realignment of the group as a
subphylum. Although, in formal terms, it is now phylum Mucoromycota that
shared a most recent common ancestor with Dikarya, the Glomeromycotina was
the first lineage of Mucoromycota to diverge (Spatafora et al.,
2016). |
Note the microsporidia and Rozella (Cryptomycota)
branches in Fig. 10, which come out as basal to all other fungi in this
analysis. Rozella, a genus of chytrid that is parasitic on other
Chytridiomycota, seems to be one of the most primitive fungi.
Microsporidia, which are parasites of animals, seem to be
derived from an endoparasitic chytrid ancestor similar to Rozella, on
the earliest diverging branch of the fungal phylogenetic tree.
The fungi, animals and plants are the only three eukaryotic
kingdoms of life that developed multicellular tissues in terrestrial
environments. They are thought to have diverged from each other roughly one
billion years ago. This study continues to support the view that the ancestors
of fungi were simple aquatic cells with flagellated spores, similar to current
chytrids. What it changes is the idea that there was a single loss of the
chytrid flagellum as terrestrial fungi diversified. Rather, the study argues for
at least four independent losses of the flagellum during early evolution of
Kingdom Fungi, coinciding with the evolution of new mechanisms of spore
dispersal.
Estimating the historical time of appearance of the major
fungal groups remains a major problem, though whole genome analyses are
beginning to aid understanding (Reynolds et al., 2017). A
significant contribution to the problem is that horizontal gene
transfer has been a significant factor in fungal evolution
(Richards et al., 2011) and, of course, fungi have been so
successful in the billion years or so of their existence.
Today’s fungal kingdom is arguably the most abundant and
diverse group of organisms on Earth. Fungi are found in every terrestrial
ecosystem as mutualist partners, pathogens, parasites, or saprotrophs. As we
mentioned in Section 1.7 above, it
is estimated that the kingdom contains 2.2 to 3.8 million species
(Hawksworth & Lücking, 2017), but only about 3 to 8% of these have been
described. If most of the unknowns are members of the traditional taxa, then
current phylogenetic inferences will be unchallenged by additional
discoveries. However, novel fungal groups could be awaiting discovery by
DNA-based environmental sampling, which is already starting to reveal
microscopic, undescribed, and unculturable fungi. Because unknowns are
unknowns, we can’t predict how such discoveries might affect our
understanding of fungal origins and evolution. A quotation we’d like to
associate with this summary is: ‘...evidence accumulates to support the
long-held view that the history of fungi is not marked by change and
extinctions but by conservatism and continuity...’ (Pyrozynski, 1976). In
other words, fungal evolution is based on the principle: if it works...don’t
fix it. Our current understanding of the broad sweep of fungal evolution is
summarised in the next Resources Box.
Resources
Box
A summary of fungal evolution
The phylogenetic tree
in this resource summarises current ideas about the broad sweep of
fungal evolution, and puts it into context by showing some markers of
geological time and animal and plant evolutionary features.
CLICK HERE to visit this resource page. |
An interesting development in the first few years of the
21st century was a trend to suggest that the first terrestrial eukaryotes
might have been fungal. A few titles will illustrate this: ‘Terrestrial
life – fungal from the start?’ (Blackwell, 2000); ‘Early cell
evolution, eukaryotes, anoxia, sulfide, oxygen, fungi first (?), and a Tree
of Genomes revisited’ (Martin et al., 2003); and ‘Devonian
landscape heterogeneity recorded by a giant fungus’ (Boyce et al.,
2007). For mycologists this was certainly a refreshing development because,
prior to this, fungi have always been ignored when theorists pondered the
origin and early emergence of life on this planet. The complete version of
what we like to call ‘The Mycologists’ Tale’ appeared in
the book entitled
Fungal Biology in the Origin and Emergence of Life
(Moore, 2013).
This book offers a new interpretation (illustrated here in
Fig. 11) of the early radiation of eukaryotes based on the emergence of
major innovations in cell biology that apply uniquely to present day fungi.
These emphasised: increasingly detailed management of the positioning and
distribution of membrane-bound compartments (vacuoles, vesicles and
microvesicles) by the filamentous components of the cytoskeleton
(microfilaments, intermediate filaments and microtubules); culminating, as
far as filamentous fungi are concerned, with emergence of the Spitzenkörper
and apical hyphal extension. These features of present day fungi are
described in detail elsewhere in this book (Chapter 5, Sections 5.10 and
5.11) and we will reference where we describe other features as we mention
them.
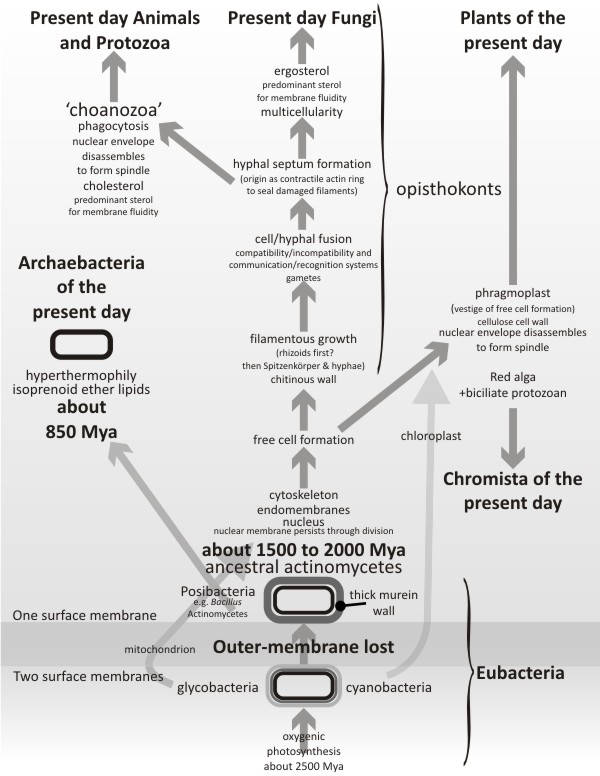
Fig. 11. The mycologist’s tree of life. The lower part of this diagram
is based on Cavalier-Smith’s tree of life (Cavalier-Smith, 2010a; his
Fig. 6), which emphasises major evolutionary changes in membrane
topology and chemistry, except that the most ancient bacteria are
shown here to be heterotrophic descendants of LUCA (the last universal
common ancestor of all current life on Earth). Eukaryotes diverge from
actinobacterial ancestors about 1,500 Mya (million years ago) and the
bulk of this illustration deals with eukaryote evolution. The most
ancient stem eukaryotes are considered to exhibit characteristics that
would today be thought of as applying to primitive fungi. Their
evolution emphasises increasingly detailed management of the
positioning and distribution of membrane-bound compartments (vacuoles,
vesicles and microvesicles) by the filamentous components of the
cytoskeleton (microfilaments, intermediate filaments and
microtubules); culminating, as far as filamentous fungi are concerned,
with emergence of the Spitzenkörper and apical hyphal extension.
Uniquely among present day eukaryotes, the fungi maintain their
nuclear membrane intact as the nuclear division progresses. The
subsequent evolution of Kingdom Fungi is outlined in
the
Resources Box above.
|
The interpretation suggests that the last universal common
ancestor of all current life on Earth (usually known by the acronym LUCA)
was a heterotrophic, mesophilic prokaryote, essentially a ‘bacterial’ cell with
the cell enveloped by two distinct lipid bilayer membranes. The earliest
prokaryotes used prebiotically synthesised organic carbon compounds as nutrients
but, as these supplies diminished, they were outstripped by the anoxygenically
photosynthetic Chlorobacteria as the most primitive surviving prokaryotic
phylum. This interpretation follows the deep phylogeny of the tree of life
published by Cavalier-Smith (2006, 2010a & b), who argued that thermophiles
evolved late, making Archaebacteria the youngest bacterial phylum and the
sisters, rather than ancestors of eukaryotes (in contrast to the interpretations
described in Section 2.4 above), which themselves diverged from actinobacterial
ancestors. Eukaryotes are generally thought to have appeared about 1.5 billion
years ago, so, for a total of about 2 billion years the only living organisms on
the planet were prokaryotes together, presumably, with their associated viruses.
CLICK HERE to download the full text
PDF of our article entitled 'A fungal root for the eukaryote tree'.
The primitive eukaryotic stem featured primitive nuclear
structures (including the nuclear membrane remaining intact as the nuclear
division progresses, which is a unique characteristic of present day
fungi) (Chapter 5, Section 5.7), added the mitochondrion by
enslavement of a bacterium (Chapter 5, Section 5.10); and evolved those aspects
of the endomembrane system and cytoskeletal architecture that are also unique
characteristics of present day fungi, in the following probable temporal
sequence:
-
Free cell formation, (Chapter 3) by
managing positioning of wall- and membrane-forming vesicles to enclose
volumes of cytoplasm to subdivide sporangia into spores, with adoption of a
chitinous cell wall, possibly as an adaptation of muramopeptide
oligosaccharide synthesis from the wall of an actinobacterial ancestor. This
is a possible branch point to plants if the phragmoplast is assumed to be a
vestige of free cell formation and the cell wall was adapted to be a polymer
of glucose rather than N-acetylglucosamine, possibly for economy in usage of
reduced nitrogen in organisms abandoning heterotrophy. Plants also evolved a
means to disassemble the nuclear envelope to form the division spindle.
-
Filamentous growth, (Chapter 4) first to
make rhizoids then apically-extending with the Spitzenkörper as the
organising centre for hyphal extension and morphogenesis to make nucleated
hyphae to explore and exploit the then extant biofilm and terrestrial debris
that had accumulated during 2 billion years of prokaryote growth, life and
death.
-
Hyphal/cell fusion, (Chapters 4 and 5,
especially Section 5.16) with associated cytoplasmic (vegetative) and
nuclear (sexual) compatibility/incompatibility systems, hypha to hypha
communication/recognition systems, autotropism, gravitropism, and
intrahyphal communication using secondary metabolites, including the
evolution of gametes.
-
Hyphal septum formation, (Chapter 4,
Section 4.12; and Chapter 5, Section 5.17) initially dependent on a
contractile ring of actin to seal the membrane of damaged filaments rapidly,
later developing ingressive wall synthesis to strengthen the seal, and
ultimately cross-wall formation at regular intervals to initiate
multicellular development (Naranjo-Ortiz
& Gabaldón, 2020). Possibly combined with the
(accidental?) fixation on ergosterol as the quantitatively predominant
sterol involved with controlling membrane fluidity in fungi.
This last is a possible branch point from chytrid level fungi to
animals (of the sort presently called choanozoa). The animal stem gradually lost
its wall and adapted cytoskeletal organisation/vesicle trafficking originally
used in wall synthesis to the new function of phagocytosis. Animals also
developed disassembly of the nuclear envelope as the division spindle forms; and
adopted cholesterol as the predominant sterol for membrane fluidity, as well as
equatorially contractile cell division. Ivarsson et al. (2020) also
argue, in their ‘alternative view on hyphal development’, that septal formation
was an important step in the evolution of multicellularity in fungi, but they
place the events ‘in the deep biosphere’. We do not see the necessity of
removing the evolution of filamentous hyphae to ‘the deeps’ from surface
biofilms and believe that most of the points discussed by Ivarsson et al.
could equally well apply to biofilms on rock surfaces (Moore, 2013).
Through this sequence of events filamentous fungi emerged 1.5
billion years ago as the first crown group of
eukaryotes. They emerged to exploit the components of the biofilms in which they
lived and the debris left by 2 billion years of prokaryote growth, and they’ve
been cleaning up the planet ever since (Moore, 2013; and see Halbwachs, 2019b).
Finally, to illustrate the ancient
importance of fungi, and maybe suggest something that accounts for their success
through the rest of geological time we offer a few quotations, which relate to
the Permian-Triassic (P-Tr) extinction event that occurred approximately 251
million years ago. The evolution of life on Earth has been interrupted by
several mass extinction events. The P-Tr event, informally known as the Great
Dying, was the Earth’s most severe extinction event (so far!), with about 96% of
all marine species and 70% of terrestrial vertebrates becoming extinct. This
catastrophic ecological crisis was triggered by the effects of severe changes in
atmospheric chemistry arising from the largest volcanic eruption in the past 500
million years of Earth’s geological history, which formed what are now known as
the Siberian Traps flood basalts. When first formed these are thought to have
covered an area in Siberia about the size of the present
continent of
Australia.
Plants suffered massive extinctions as well as animals:
‘…excessive dieback of arboreous vegetation, effecting destabilisation and
subsequent collapse of terrestrial ecosystems with concomitant loss of standing
biomass...’ occurred ‘throughout the world’.
However, the result of all this death and destruction is that
‘…sedimentary organic matter preserved in latest Permian deposits is
characterised by unparalleled abundances of fungal remains, irrespective of
depositional environment (marine, lacustrine [= lake sediments], fluviatile
[=river/stream deposits]), floral provinciality, and climatic zonation.’ The
quotations were taken from Visscher et al. (1996).
The Cretaceous-Tertiary (K-T) extinction of 65 million years ago
is another one that we all know a little bit about, because it was caused by a
meteor collision that caused the Chicxulub crater in Mexico and is blamed for
the extinction of the dinosaurs. The K-T boundary is characterised by high
concentration of the element iridium, which is rare on Earth but common in space
debris, such as asteroids and meteors. Current understanding is that a meteor
hit the Earth at the end of the Cretaceous and the iridium-rich layer
was resulted as the
world-wide dust cloud produced by the impact settled to the
ground. As the Cretaceous is the last geological period in which dinosaur
fossils are found, the belief is that the meteor collision at Chicxulub caused
the extinction of the dinosaurs. There was also widespread deforestation right
at the end of the Cretaceous, which is assumed to be due to post-impact
conditions of high humidity (caused by widespread rain), decreased sunlight and
cooler global temperatures resulting from increased atmospheric sulfur aerosols
and dust (and see our discussion of patterns of evolution of mushrooms in
Section 3.8).
However, coincident with all this death and destruction of animal and plant
life at the K-T boundary there is a massive proliferation
of fungal fossils. Vajda & McLoughlin (2004) put it like this: ‘…This fungi-rich
interval implies wholesale dieback of photosynthetic vegetation at the K-T
boundary in this region. The fungal peak is interpreted to represent a dramatic
increase in the available substrates for [saprotrophic] organisms (which are not
dependent on photosynthesis) provided by global forest dieback after the
Chixculub impact.’
So, it is the same story as at the P-Tr extinction boundary: while the rest
of the world was dying, the fungi were having a party! But that might not be the
full significance of this anecdote, because Casadevall (2005) suggests that the
massive increase in the number of fungal spores in the atmosphere of the time
caused fungal diseases that ‘…could have contributed to the demise of dinosaurs
and the flourishing of mammalian species…’ The impact of fungi on our own
origins is as great as their impact on the world habitat.
Updated September, 2020
|